Global Site
Displaying present location in the site.
High-Capacity Backbone Networks and Multilayer Integrated Transport Systems
Vol.10, No.3 July 2016, Special Issue on Telecom Carrier Solutions for New Value CreationExponentially increasing communications traffic and the fast-growing popularity of cloud services is putting tremendous stress on backbone networks, forcing telecom operators to boost the capacity of their networks and to introduce flexible transport systems capable of adapting to continuously changing network demands. At the same time, it has been desired as social network infrastructure that systems are reliable enough to ensure uninterrupted communications in the face of wide-area disasters. To address these needs, NEC has been developing technologies that support high-capacity, high-reliability networks. This paper discusses the various technologies developed and the latest network system based on these technologies, and also indicates future approaches.
1. Introduction
The rapid popularization of high-definition movie distribution services and social networking services in recent years has led to the need for an increase in the capacity of the transport systems that support telecommunication backbone networks. At the same time , there is growing demand for robust systems with high fault tolerance that can provide uninterrupted communications under even the most adverse conditions, while keeping capital expenditures (CAPEX) and operating expenditures (OPEX) to a minimum, to provide the communication services as one of most critical form of social infrastructure.
To address these issues, NEC has been proceeding with the deployment of solutions using high-capacity optical communication technology and high-capacity switching technology composed of layers of wavelength division multiplexing (WDM) in L0, optical transport network (OTN) in L1, and packet in L2, as well as transport software-defined networking (SDN).
This paper discusses the technologies developed by NEC aimed at building high-capacity, high-reliability optical networks and the SpectralWave DW7000 - multilayer integrated transport system.
2. Configuration of the Multilayer Integrated Transport System
The configuration of multilayer integrated transport system is shown in Fig. 1. This system is comprised of optical switch and OTN switch, as well the packet switch component.
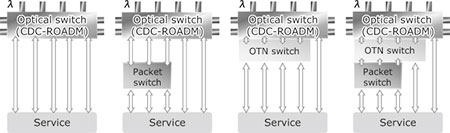
The optical switch (L0), OTN switch (L1), and packet switch (L2) components can be integrated or operated independently. Optimum configurations can take different forms depending on the scale of the network and the applications used. This makes it possible to select paths on a multilayer basis from L0 to L2, enabling construction of economical networks with efficient layout of network resources.
3. Technologies to Achieve High-Capacity, High-Reliability Backbone Networks
3.1 Super-channel Transmission and Spectrum-shaping Technology
Conventionally, to increase the capacity of a WDM system, the transmission rate per wavelength is increased. However, in order to achieve a transmission rate over 100 Gbps per wavelength, the most effective technique is to use a super-channel transmission technology that bundles multiple carriers for transmission. The use of multiple carriers makes it possible to increase capacity while maintaining a transmission distance that's roughly the same as a conventional system.
Also, by applying this technique in combination with spectrum shaping technology called Nyquist shaping, spectrum efficiency can be improved since the occupied bandwidth per wavelength, which normally has a width of 50 GHz (with 100G DP-QPSK), can be reduced by 25% to a width of 37.5 GHz.
3.2 Optimization of the Interval between Signal Wavelengths (Flexible-grid)
In a conventional WDM transmission system, optical signals are laid out on a fixed grid that divides the signal waveband at uniform intervals (usually 50 GHz intervals). Thus, as shown in Fig. 2 (b), even when Nyquist shaping reduces the occupied frequency bandwidth of the optical signals, unused frequency bands cannot be utilized. Nor is it possible for a WDM system equipped with a fixed-grid to transmit signals when multiple carrier waves have been bundled at high density.
However, by making the signal wavelength layout grid finer than the conventional fixed-grid (in other words, by creating a flexible-grid) optical signals which Nyquist shaping has been applied and super-channel signals can be allocated to optimal wavelength intervals. An image of optimized signal wavelength intervals after the application of Nyquist shaping is shown in Fig. 2 (c). Optimization of the signal wavelength intervals can help improve total transmission capacity per fiber by about 33%.
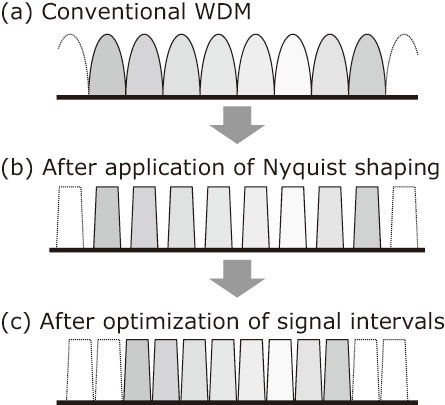
3.3 LO Selection (Filterless)
Optical filters such as arrayed waveguide grating (AWG) and wavelength selective switch (WSS) filters are usually deemed necessary when the received WDM signal is connected to a transponder. However, transponders can receive the desired signal without using an optical filter (filterless) by tuning the local oscillator (LO) light inside the transponder to the desired reception wavelength and performing coherent detection.
Optimization of LO selection control makes it possible to reduce both the power consumption and size by 50% compared to when an optical filter is used. Also, in super-channel transmission, the increased transmission penalty caused by optical filter narrowing effects at reconfigurable optical add/drop multiplexer (ROADM) nodes will be conspicuous. However, by using LO selection to remove the optical filter, you can achieve long-distance transmission while minimizing the transmission penalty.
3.4 Multilayer Design Technology and Third Route Switching
To design a highly efficient, highly reliable network, you need a system configuration that is optimal in multiple layers. By using cost simulation to ensure an economical design, as well using different routing of lines in protection configuration to ensure reliability, and by taking into account switching to backup lines (third routing) in the event of a wide-area disaster, optimal multilayer networks can be achieved. An example of the multilayer design that takes these into account is shown in Fig. 3.
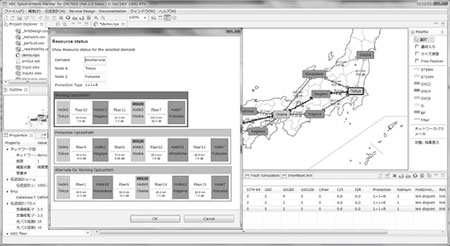
Once designed, the multilayer paths are registered and managed in a network management system (NMS). When the network is made up of optical switching nodes that use the colorless, directionless reconfigurable optical add/drop multiplexer (CDC-ROAMDM), optical paths can be switched from a remote NMS - which normally can only be achieved by altering the fiber connection at the on-site station. Moreover, by registering the third routing - which will function as backup lines - in the NMS, the services can be automatically switched to the third route in the event of a wide-area disaster, enabling quick and easy arrangement of detours. Also, by executing switching commands to the third route from the NMS under centralized monitoring, you can control the switching - which makes it easier to envision the current routing and is faster than restoring the autonomous distributed control system. Fig. 4 shows the switchover to the third routing while the devices and NMS work in cooperation.
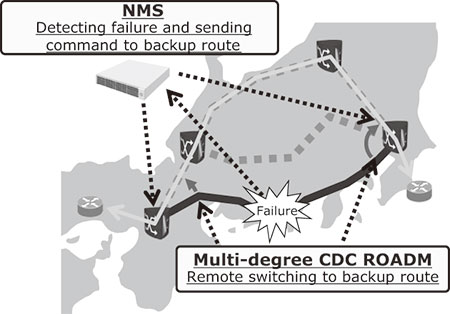
4. Features of the SpectralWave DW7000
NEC has applied the technologies discussed above to SpectralWave DW7000 multilayer transport system, which has been deployed by telecom carriers around the world. Employing a 400 G super-channel transmission method and carrying signals of up to 204 Tbps (64 waves X 400 Gps X 8 degrees = 204.8 Tbps), this transport system enables transmission over distances of more than 3,000 km. It also incorporates a platform that can be applied to 1 Tbps super-channel transmission in the future. The main specifications are shown in Table, while its external appearance can be seen in Photo.
Table Main specifications of the SpectralWave DW7000.
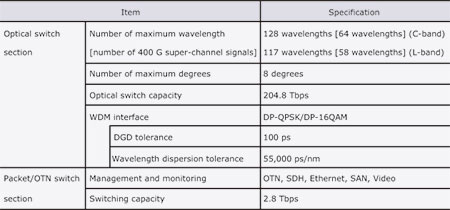
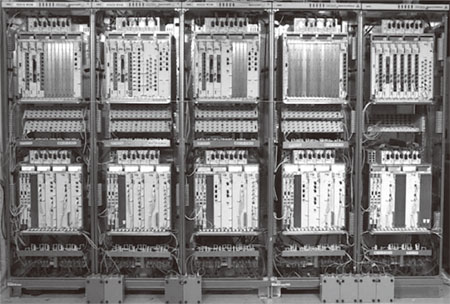
5. Commitment to the Transport SDN
The architecture of conventional transport systems is built on a premise of static operation. However, the popularization of data-rich services such as cloud computing, has caused an increase in mesh-shaped traffic patterns between servers (interconnection between data centers), making the total volume of traffic and its chronological fluctuation range greater than previously. This has made it necessary to dynamically vary the configuration of transport networks, as well. Moreover, the two biggest challenges telecom carriers are now facing - namely, reduction of CAPEX and OPEX and creation of new sources of income - need to be solved at the same time.
Against this background, NEC has defined the following three goals to enhance the value of new transport systems.
1) Reduction of total cost of ownership (TCO)
- Reduction of CAPEX by taking advantage of general-purpose servers and open sources and by optimizing network resources
- Reduction of OPEX by simplifying provisioning, system launching, and maintenance
2) Create New values on network and increased revenue
Using optimal networks (service slices) according to services, the network will meet end user requirements and lead to new sources of revenue.
3) Achievement of flexible networks that allow the addition of new services through end-toend (E2E) service orchestration
Siloed management using existing networks will be changed to operation and management in E2E service domains.
To achieve these requirements, NEC proposes the Transport SDN, in which the SDN technology is applied to transport networks.
The world of networks achieved by the NEC's Transport SDN is shown in Fig. 5. In this network, the E2E service orchestrator virtually separates the units of services that effectively utilize physical network resources. This makes it possible to generate service slices on an on-demand basis according to the network requirements of end users, enabling telecom operators to offer newly added value. At the same time, this will contribute to the simplification of operation and management through full utilization of the E2E service orchestrator and to the reduction of TCO through effective use of network resources.
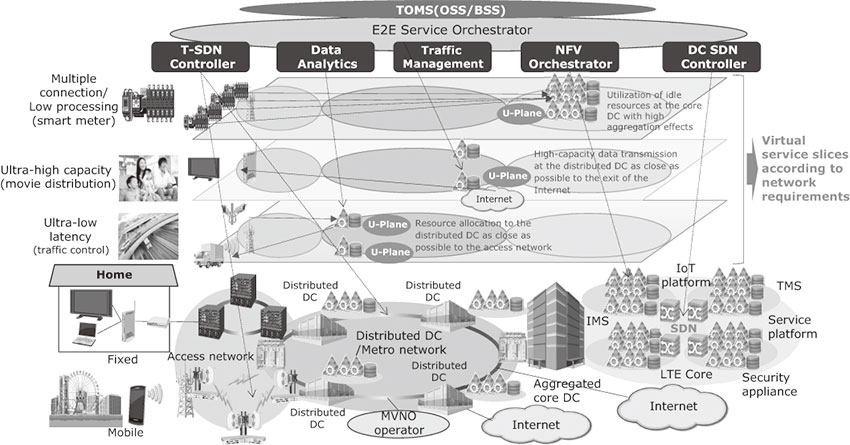
6. Conclusion
This paper has shown how NEC's technologies can achieve high-capacity, high-reliability backbone networks that can flexibly cope with wide-area disasters, with a focus on SpectralWave DW7000 multilayer in tegrated transport system - in which these component technologies have been applied.
To better address recent requirements for dynamic alteration in transport network configuration, NEC plans to introduce to its clients the multilayer integrated transport system, which features a flexible configuration adaptable to a wide variety of rapidly changing requirements. Together with the proposed Transport SDN, this will allow our customers to choose the optimal solution for their needs.
Authors' Profiles
Converged Network Division
Manager
Converged Network Division
Manager
Converged Network Division
Manager
Converged Network Division
Manager
Converged Network Division
Manager
Converged Network Division